- 1Diabetes Research Program, Holman Division of Endocrinology, Diabetes and Metabolism, Department of Medicine, New York University School of Medicine, New York, NY, United States
- 2Applied Therapeutics Inc, New York, NY, United States
Human skin plays an important role protecting the body from both extrinsic and intrinsic factors. Skin aging at cellular level, which is a consequence of accumulation of irreparable senescent keratinocytes is associated with chronological aging. However, cell senescence may occur independent of chronological aging and it may be accelerated by various pathological conditions. Recent studies have shown that oxidative stress driven keratinocyte senescence is linked to the rate limiting polyol pathway enzyme aldose reductase (AR). Here we investigated the role of three novel synthetic AR inhibitors (ARIs) AT-001, AT-003 and AT-007 in attenuating induced skin cell senescence, in primary normal human keratinocytes (NHK cells), using three different senescence inducing agents: high glucose (HG), hydrogen peroxide (H2O2) and mitomycin-c (MMC). To understand the efficacy of ARIs in reducing senescence, we have assessed markers of senescence, including SA-β-galactosidase activity, γ-H2AX foci, gene expression of CDKN1A, TP53 and SERPINE1, reactive oxygen species generation and senescence associated secretory phenotypes (SASP). Strikingly, all three ARIs significantly inhibited the assessed senescent markers, after senescence induction. Our data confirms the potential role of ARIs in reducing NHK cell senescence and paves the way for preclinical and clinical testing of these ARIs in attenuating cell aging and aging associated diseases.
Introduction
Skin aging is a complex process that is governed by key contributions from several factors (Farage et al., 2008). Gender, ethnicity, genetic variations, inherited genes, radiation exposure, diabetes, nutrition, environment and lifestyle habits contribute to the process of skin aging (Farage et al., 2017; Gunn et al., 2014; Makrantonaki et al., 2012; Green et al., 2011; Tobin, 2017; McDaniel et al., 2018; Argyropoulos et al., 2016; Panich et al., 2016). The process of aging is initiated at cellular level by loss of function and ability to replenish damaged keratinocytes. This process, defined as senescence of keratinocytes, has an overall adverse impact on skin aging. The hallmark of cellular senescence is associated with increase of both cytosolic and mitochondrial reactive oxygen species production (ROS), expression of key senescence markers including SERPINE1/PAI1, cell cycle inhibitors, such as CDKN1A/p21, pro-apoptotic marker genes, including TP53/p53 and release of senescence associated secretory phenotype (SASP) (Vaughan et al., 2017; Yepuri et al., 2016). Furthermore, Histone γ-H2AX foci, sensitive marker of double-stranded DNA breaks (DSBs) and telomere shortening increases in damaged and senescent cells (Hovest et al., 2006; Sedelnikova et al., 2004; Kaul et al., 2011).
Impaired carbohydrate metabolism plays a key role in the pathogenesis of obesity, diabetes, and metabolic syndrome, and consequently associated with skin diseases (Stefanadi et al., 2018; Ness, 2004; Lakdawala et al., 2013; Cosgrove et al., 2007; Danby, 2010). Several studies (Jannapureddy et al., 2021; Vedantham et al., 2012; Ramasamy and Goldberg, 2010) have demonstrated that increase in aldose reductase (AR) enzymatic activity is associated with oxidative stress, and AR inhibition reduces ROS and advanced glycation end products, which has been linked to skin cell senescence (Jannapureddy et al., 2021; Vedantham et al., 2012; Ramasamy and Goldberg, 2010; Ananthakrishnan et al., 2009). Furthermore, in the context of skin cells, Farres and colleagues (Ruiz et al., 2011; Ruiz et al., 2009) established that AR is able to catalyze reduction of retinal to retinol in vitro. A previous study (Yepuri et al., 2016; Kang et al., 2011) demonstrated that normal human keratinocytes express AR strongly compared to other enzymes proposed to be physiologically important retinal reductases. Recently, we demonstrated the link between AR activity and senescence in subcutaneous adipose tissue of mice fed with high fat diet (Thiagarajan et al., 2022). Here, we propose that AR is a potential therapeutic target for beneficial modulation of skin cell properties and that reducing AR activity in human keratinocytes is a potential mechanism to modify keratinocyte senescence. In our present study we tested the role of potent and selective AR inhibitors (ARIs) AT-001, AT-003, AT-007 (Mylari, 2014a; Mylari, 2014b; Shendelman, 2018; Wasmuth et al., 2013; Wasmuth et al., 2014), in progression of AR induced human keratinocyte senescence.
Methods
Cell culture and treatment
Normal pooled adult Human Epidermal Keratinocytes (NHK) were obtained from PromoCell (cat # C-12006) maintained and passaged in serum free Keratinocyte complete growth medium (PromoCell, cat # C-20111). Early passage cells (P4-P6) were used for all our studies. To induce senescence and asses the effect of AT compounds on senescence phenotype we treated cells either with 200 nM Mitomycin (cat # 10107409001) for 24 h, 25 mM of D-glucose to mimic high glucose conditions and 10 µM of H2O2 for 48 h or 400 µM for 1 h followed by washout and consequently cells were analyzed for senescence markers at day 10. In treatment groups, AT compounds (AT-001, AT-003, AT-007) were added at a concentration of 0.2 nM 10 min prior to senescence induction. For all compounds, 1 mM stock solutions were prepared in DMSO, consequent dilutions to 0.2 µM stocks were prepared in PBS under sterile conditions. Vehicle also referred to as Veh which was added to control cells were prepared accordingly. The dose used is calculated based on EC50 for all AT compounds (Shendelman, 2018; Wasmuth et al., 2013; Wasmuth et al., 2014).
Human AR activity assay
Colorimetric assessment of human AR activity was performed using AR Activity Kit (BioVision, Inc. cat # K369) as per manufacturer’s instructions. Absorbance was measured at 340 nm for a duration of 60 min at a regular time interval of 90 s using TECAN Infinity Pro 200 plate reader. Calculations were performed as per manufacturer’s instructions. The resultant data was normalized to total protein, which was assessed using Pierce™ BCA Protein Assay Kit (Thermo Scientific™ cat # 23225).
Senescence associated β galactosidase staining (SA-β-gal)
NHK cells were plated in standard six well plates. SA-β-gal staining was performed using Cellular Senescence Staining Kit obtained from Cell Biolabs, Inc. (cat# CBA230) as per manufacturer guidelines. In brief, cells were washed and fixed by the fixative provided by the kit. Cells were incubated with SA-β-gal staining solution for 16 h. Cells were counter stained with Syto-13 (ThermoFisher Scientific cat# S7575) dye to obtain accurate cell count. Alternatively, florescent based approach was used to measure cellular senescence using CellEvent™ Senescence Green Detection Kit from ThermoFisher Scientific (Cat# C10851). The experiment was performed as per manufacturer’s guidelines. Cells were counter stained with DAPI to obtain accurate cell count. Images were taken at ×10 magnification using Ziess Axio-observer light and florescent microscope. For CellEvent™ Senescence Green Detection Kit, images were taking at 488 nm filter set. Cell count was performed using NIH ImageJ software.
Cytosolic and mitochondrial superoxide measurements
NHK cells were plated in 12 wells plates. Post respective treatments, cells were stained with live cell superoxide indicators. For detecting cytosolic superoxide, cells were incubated with 5 µM dihydroethidium (ThermoFisher Scientific Inc. cat # D1168) for 30 min. To detect mitochondrial superoxide, cells were incubated with 5 µM MitoSOX dye (ThermoFisher Scientific, cat# M36008) for 10 min. Cells were later washed with PBS to remove excess dye and fixed with 3.7% paraformaldehyde (PFA). Imaging was performed at ×10 magnification using EVOS XL Core Imaging System. Relative intensity for images was calculated using NIH ImageJ software.
Quantitative PCR
RNA from treated cells was extracted using RNeasy Plus Mini Kit (QIAGEN, cat#74134) and cDNA was synthesized using qScript™ cDNA SuperMix (Quanta BioSciences, Inc. cat # 95048). RT-qPCR was performed using Taqman gene expression assays. 20 ng of RNA input per reaction was used for all qPCR studies. Amplification reactions were performed by Applied Biosystems 7500 Fast Real-Time PCR System. Relative fold change in expression for desired genes was performed using the ΔCT method of analysis. All gene expression data were normalized to housekeeping gene PPIA. Genes used to determine expression are listed in Table 1.
ELISA for cytokine measurements
ELISA was performed to determine cytokine release into Condition medium. Condition medium at day 10 was collected from skin cells treated H2O2 as described above. ELISA was performed for cytokines released in condition medium for IL1β (Abcam, cat # ab214025), TNFα (Abcam, cat # ab181421), MCP1 (Abcam, cat # ab179886) and IL6 (Abcam, cat # ab178013) as per manufacturers protocols. All data was normalized to total protein content assessed by BCA assay. Absorbance as described in the protocol was measured using TECAN infinity pro 200 plate reader.
Gamma H2A.X staining
To measure DNA damage in NHK cells treated with long term H2O2, Gamma H2A.X Staining Kit (ABCAM; cat # ab242296) was used. Cells were stained according to company protocol followed by florescent imaging using EVOS XL Core Imaging System at ×10 magnification. Relative intensity and cell count for images was calculated using NIH ImageJ software.
Statistical analysis
All data was analyzed using GraphPad PRISM 9 software (GraphPad, LaJolla, CA). n represents biological replicates obtained from two consecutive batches performed at two different time points with cells originating from separate source. Each batch comprised of n = 2 obtained from two separate culture dishes or wells. All data are expressed as mean ± SEM. Unpaired t-test was performed to compare data between two groups and Ordinary one-way ANOVA with Tukey’s multiple comparisons test was implemented for comparison between more than two groups. p < 0.05 was considered statistically significant between groups.
Results
AR activity is increased in senescent human keratinocytes
Previous studies have demonstrated the deleterious effect of the activity of the polyol pathway in accelerating keratinocyte senescence (Kang et al., 2011). To determine if senescence is linked to changes in AR activity, we induced senescence by treating human keratinocytes either with mitomycin C (MMC), H2O2 or high glucose (HG) and measured AR activity. We observed a 3-fold increase in AR activity upon treatment with 10 µM H2O2 or 25 mM D-glucose compared to controls (Figures 1A, B) and a 2.5-fold increase upon treatment with 200 nM MMC (Figure 1C). These data link increases in activity of polyol pathway enzyme AR in keratinocyte senescence.
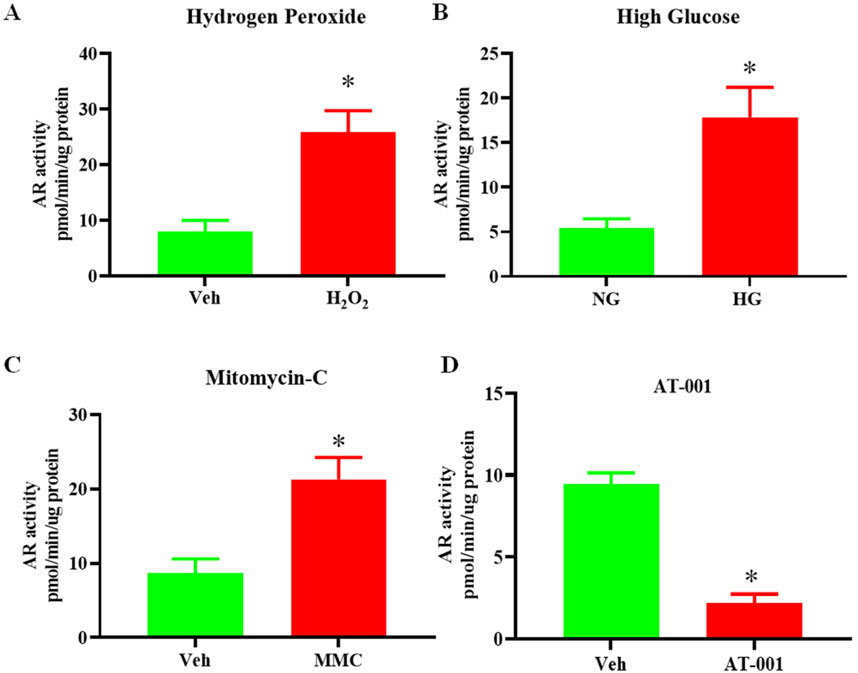
Figure 1. Senescence induction upregulates AR activity. AR activity measured by colorimetric assay in human keratinocytes either treated with (A) 10 uM H2O2 or Vehicle (Veh- dH2O) (B) 25 mM high glucose (HG) or normal glucose (5 mM) (NG) for 48 h (C) 200 nM Mitomycin-C (MMC) for 24 h compared to Veh (DMSO) and (D) 0.2 nM AT-001 for 48 h vs. Veh (1:5,000 dilution DMSO in PBS). Data are presented as the mean ± SEM. n = 4 per group obtained from two consecutive experiments. Unpaired t-test was implemented for comparison between two groups. p* < 0.05 vs. Veh or NG.
ARIs prevent HG induced senescence
The main focus of our study is to test the preventive role of three different, novel and highly-selective ARIs, AT-001, AT-003 and AT-007 in induced keratinocyte senescence. We initially tested if AT-001, which is the parent drug for AT-003 and AT-007 can inhibit AR activity in normal keratinocytes and our data clearly demonstrated more than 75% reduction in AR activity upon AT-001 treatment compared to vehicle treatment (Figure 1D). SA-β-gal staining, gene expression of CDKN1A, TP53 and SERPINE and both cytosolic and mitochondrial (mito) ROS superoxide production are well-established gold standard markers for cellular senescence. To test our hypothesis, we measured these key markers to confirm the preventive role of ARIs on human keratinocyte senescence. As it was evident that all three senescence-inducing agents significantly increased AR activity, we next wanted to investigate if ARIs prevented HG induced senescence. Treatment with HG for 48 h induced senescence by 30% as demonstrated by SA-β-gal staining (Figure 2A). Treatment with all three compounds 10 min prior to addition of HG significantly prevented HG induced senescence, of which AT-001 and AT-003 demonstrated maximum inhibition. Morphology observed by cell tracker dye Syto-13® displayed fewer flattened cells (typical morphology of senescent cells) in ARI treatment groups compared to the control vehicle-treated HG group (Figure 2A). We next confirmed significant downregulation of HG increased gene expression CDKN1A, TP53 and SERPINE by all three ARIs (Figure 2B). Furthermore, HG induced cytosolic and mito superoxide generation, which was measured by DHE and MitoSOX dye respectively was also strongly inhibited by AT-001, AT-003 and AT-007 (Figures 2C, D). Our data above provides strong evidence and emphasizes the importance of these compounds in preventing HG induced cellular senescence.
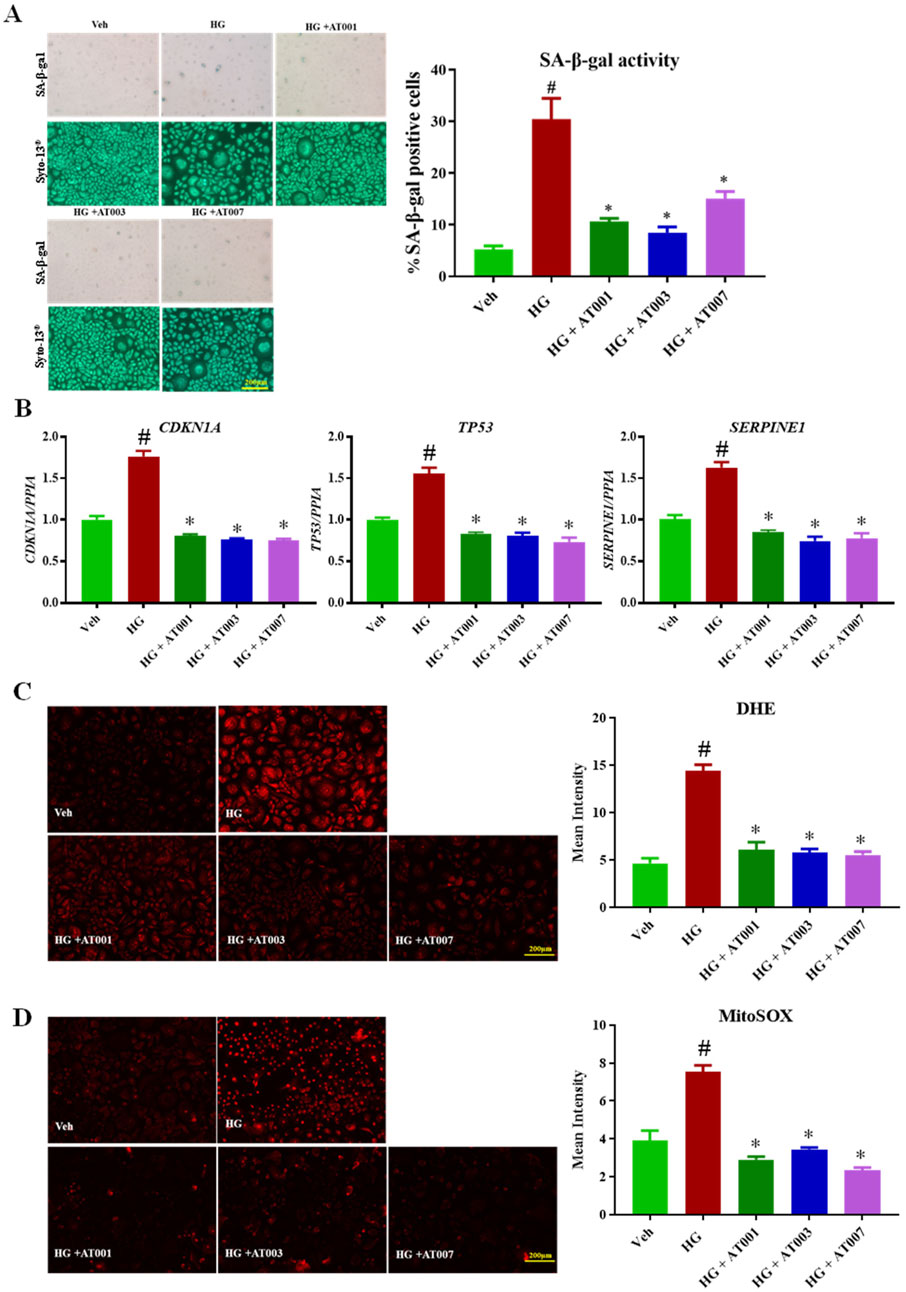
Figure 2. ARIs prevent HG induced senescence phenotype. Human keratinocytes were treated with 25 mM HG or normal condition medium NG. (A) SA-β-gal staining was performed to assess positive senescent cells. (B) qPCR analysis for confirming gene expression of senescent markers CDKN1A, TP53 and SERPINE1. (C) Skin Cells stained with 5 µM DiHydroethidium (DHE) dye for 30 min to detect cytosolic superoxide and (D) cells stained with 5 µM MitoSOX dye for 10 min to detect mito superoxide. Data are presented as the mean ± SEM. n = 4 per group obtained from two consecutive experiments. Ordinary one-way ANOVA with Tukey’s multiple comparisons test was implemented for comparison between groups. p* < 0.05 vs. NG. p# < 0.05 vs. Veh.
ARIs prevent hydrogen peroxide induced senescence
H2O2 is a well-known chemical agent, which causes senescence and increases reactive oxygen species generation in cells (Ramis et al., 1989). We next tested the efficacy of ARIs in preventing H2O2 induced senescence phenotype. Exposure to 10 µM H2O2 for 48 h significantly upregulated all the key markers of senescence tested with a 3-fold increase in SA-β-gal positive keratinocytes (Figure 3A). Treatment with the ARI compounds 10 min prior to addition of H2O2 significantly inhibited H2O2 induced senescence phenotype of which AT-001 completely abolished H2O2 induced SA-β-gal positive staining (Figure 3A). H2O2 induced DHE and MitoSOX staining was significantly inhibited by AT-001, AT-003 and AT-007 (Figures 3B, C). Gene expression of CDKN1A, TP53 and SERPINE was consistently downregulated by all three ARIs (Figure 3D). AT-001 is the parent drug for other derivatives AT-003 and AT-007. We next wanted to investigate the efficacy of AT-001 on long term treatment of H2O2. As described in the methods, keratinocytes were initially treated with 400 µM H2O2 for 1 h with or without AT-001 and the cells were allowed to be incubated in AT-001 for 10 days. At day 10 we observed a strong increase in fluorescence signal in cells treated only with H2O2 compared to vehicle treated group for γ-H2AX stain which is a marker for DNA damage and impaired cell proliferation. The fluorescence signal was abolished in cells co-treated with AT-001 and H2O2. (Figure 3E). Alternatively, we have used florescent based SA-β-galactosidase staining approach to detect senescence in these conditions. As observed in Figure 3F, AT-001 prevented florescent senescence signal induced by long term H2O2 treatment. We then assessed the SASP release into condition medium in keratinocytes upon H2O2 treatment. We observed significantly higher release of SASP IL1β, IL6, MCP1 and TNFα in H2O2 treated cells and that treatment with AT-001 reduced the increases in SASP (Figure 3G). Long term exposure of H2O2 significantly increased gene expression of CDKN1A, TP53, SERPINE, CDKN2A and well-established keratinocyte senescence marker LMNB1 (Figure 3H), AT-001 treatment blocked the increases in all these senescent marker genes (Figure 3H).
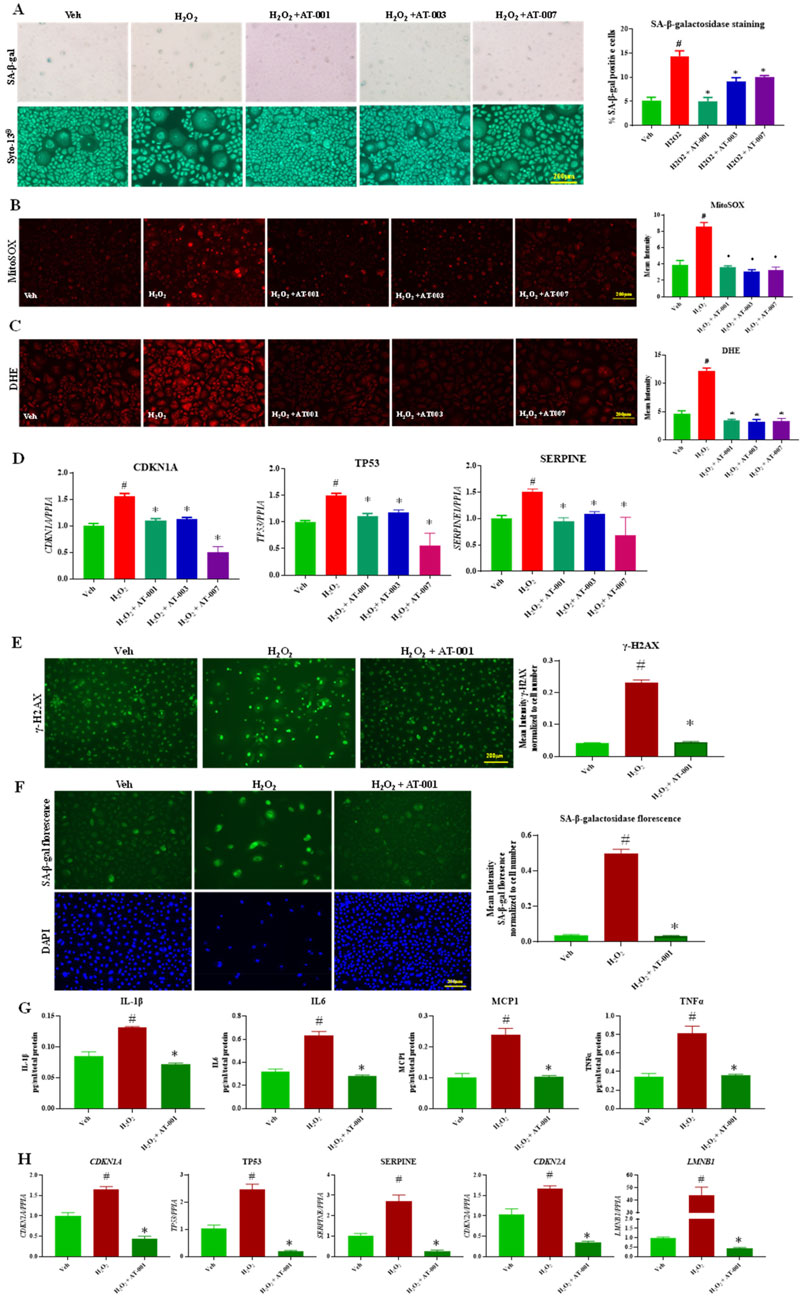
Figure 3. ARIs prevent H2O2 induced senescence phenotype. Human keratinocytes were treated with 10 µM H2O2 or Veh (dH2O) and ARIs for 48 h. (A) SA-β-gal staining was performed to assess positive senescent cells. (B) Skin Cells stained with 5 µM DiHydroethidium (DHE) dye for 30 min to detect cytosolic superoxide and (C) cells stained with 5 µM MitoSOX dye for 10 min to detect mito superoxide. (D) qPCR analysis for confirming gene expression of senescent markers CDKN1A, TP53 and SERPINE1. (E) Gamma H2A.X Staining and (F) CellEvent™ Senescence Green Detection staining in human keratinocytes treated with Veh (dH2O), 400 µM H2O2 for 1 h followed by assessment of cells at day 10 with or without ARI AT-001. (G) ELISA to measure cytokine release in condition medium collected at day 10 and (H) qPCR analysis for confirming gene expression of senescent markers CDKN1A, TP53 and SERPINE1 CDKN2A and LMNB1. Data are presented as the mean ± SEM. n = 4 per group obtained from two consecutive experiments. Ordinary one-way ANOVA with Tukey’s multiple comparisons test was implemented for comparison between groups. p* < 0.05 vs. H2O2. p# < 0.05 vs. Veh.
ARIs prevent mitomycin induced senescence
To strengthen our hypothesis, we have used a third approach which is drug induced accelerated senescence approach. Here, we treated with 200 µM of MMC for 24 h which induced approximately 35% SA-β-gal positive keratinocytes (Figure 4A). Consistent with our above finding, all three compounds significantly prevented MMC induced senescence (Figure 4A). In addition, all three compounds significantly downregulated MMC induced gene expression of CDKN1A, TP53 and SERPINE. MMC treatment induced cytosolic and mito superoxide production was consistently prevented with AT-001, AT-003 and AT-007 (Figures 4B–D). Taken together our data demonstrates that all three ARI compounds were effective in attenuating human keratinocyte senescence.
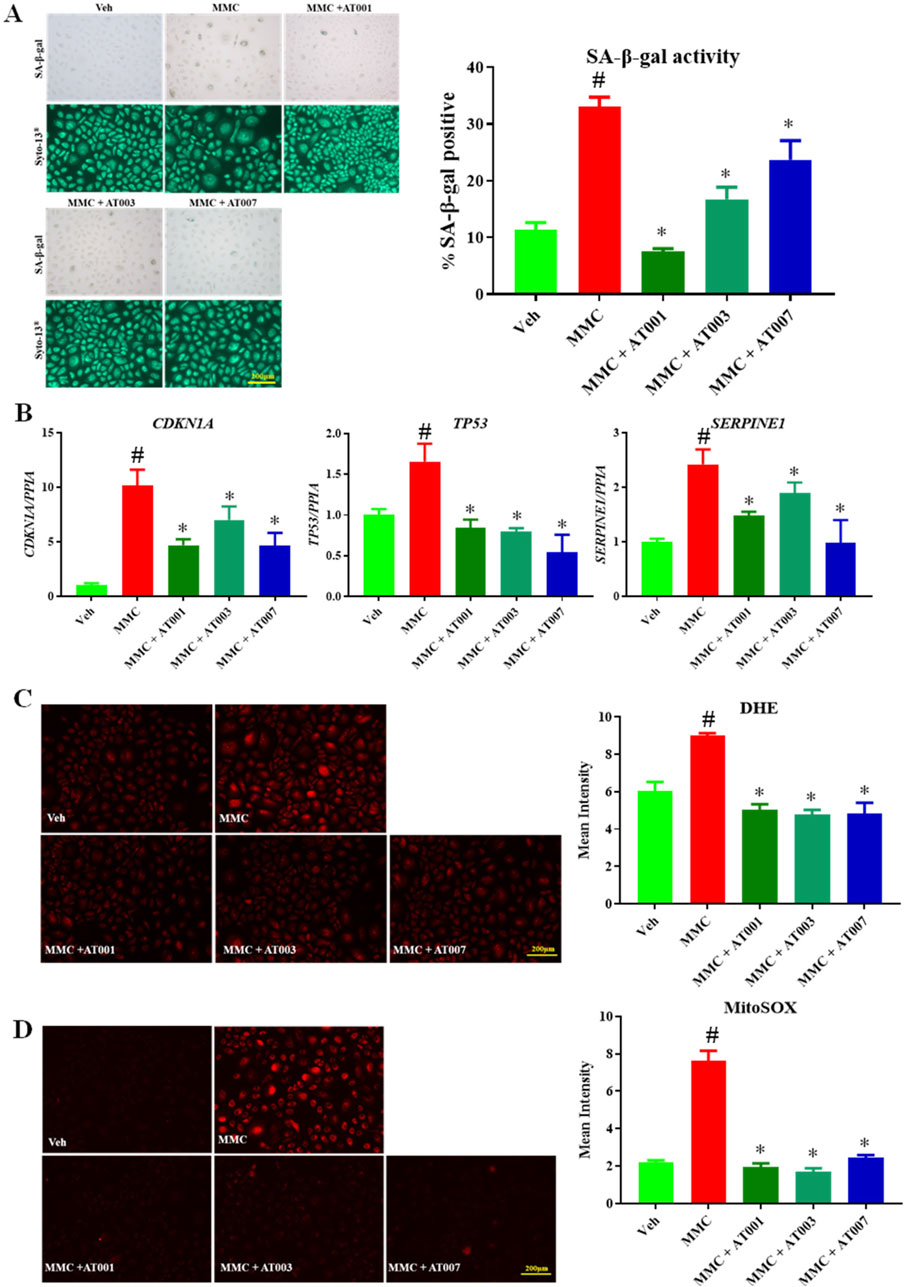
Figure 4. ARIs prevent MMC induced senescence phenotype. Human keratinocytes were treated with 200 nM MMC or Veh (DMSO) and ARIs for 24 h. (A) SA-β-gal staining was performed to assess positive senescent cells. (B) qPCR analysis for confirming gene expression of senescent markers CDKN1A, TP53 and SERPINE1. (C) Skin Cells stained with 5 µM DiHydroethidium (DHE) dye for 30 min to detect cytosolic superoxide and (D) cells stained with 5 µM MitoSOX dye for 10 min to detect mito superoxide. Data are presented as the mean ± SEM. n = 4 per group obtained from two consecutive experiments. Ordinary one-way ANOVA with Tukey’s multiple comparisons test was implemented for comparison between groups. p* < 0.05 vs. MMC. p# < 0.05 vs. Veh.
Discussion
Induction of senescence in fibroblasts by exposure to high glucose and its link to AR expression was first demonstrated by Sibbitt et al. (1989). Our previous study in adipose tissue demonstrated an increase in gene expression of AKR1B3, the mice isoform of human AKR1B1, the gene encoding for the aldose reductase enzyme at day 7 of high fat diet feeding (Thiagarajan et al., 2022). In this study, we demonstrated that increased AR activity precedes increased AR gene expression, occurring within 48 h of high glucose or H2O2 treatment, and within 24 h of MMC treatment. This is in line with prior studies demonstrating that AR activity can be regulated post-transcriptionally, and AR activity is often independent of expression upregulation (Thiagarajan et al., 2022).
This study and earlier studies in fibroblasts and adipose tissue clearly demonstrate a link between induction of senescence and AR expression (Thiagarajan et al., 2022; Sibbitt et al., 1989). However, it should be noted that a study using DNA-damage induced senescence model AR appeared to have the opposite effect on senescence (Kang et al., 2011) than what is observed in this study. This difference in AR axis in senescence is, perhaps, attributable to the DNA-damage induced vs. H2O2 and MMC induced models of senescence.
ROS generation and oxidative stress are well established mechanisms which lead to accelerated senescence phenotype (Yepuri et al., 2016; Yepuri et al., 2012). Increased AR activity has been linked to increased ROS generation and oxidative stress in multiple cell types (Jannapureddy et al., 2021; Ananthakrishnan et al., 2009; Lee and Chung, 1999; Srivastava et al., 2011; Tang et al., 2014; Tang et al., 2008; Williamson et al., 1993). In addition, our earlier studies demonstrated that flux via AR downregulates NAD + dependent SIRT1 activity and drives hyperacetylation of the transcription factor EGR1 under hyperglycemic conditions and ischemic conditions in cardiovascular cells (Vedantham et al., 2014). It is well established in the literature that SIRT1 activity and expression are key regulators of cellular senescence (Chen et al., 2020; Hwang et al., 2013; Lee et al., 2019). It is possible that AR may mediate senescence, in part, vis SIRT1 axis. Clearly, the novel AR inhibitors, AT-001, AT-003 and AT-007, are effective in reducing oxidative stress, along with downregulation of gene expression of key senescence markers, SA-β-gal activity, and SASP. Taken together, all three compounds demonstrated high efficacy in retarding senescence in human keratinocytes.
The skin, considered as the largest organ of the human body, plays an important role in protecting the body from both intrinsic and extrinsic stressors. Additionally, skin aging has broader implications for longevity and cardiometabolic complications (Franco et al., 2022). The protective role of ARI compounds in skin cell senescence have created a new direction and opportunity for testing AR inhibitors in attenuating skin aging.
Conclusion
In this study, we demonstrate that treatment of skin cells with high glucose, mitomycin-c and H2O2 induces senescence via increased AR activity (Scheme 1). Treatment of skin cells with AR inhibitors prevented oxidative stress and ROS generation and attenuated senescence markers (Scheme 1). These data indicate a potential role for potent and selective AR inhibitors, such as AT-001, AT-003 and AT-007, in attenuating skin aging.
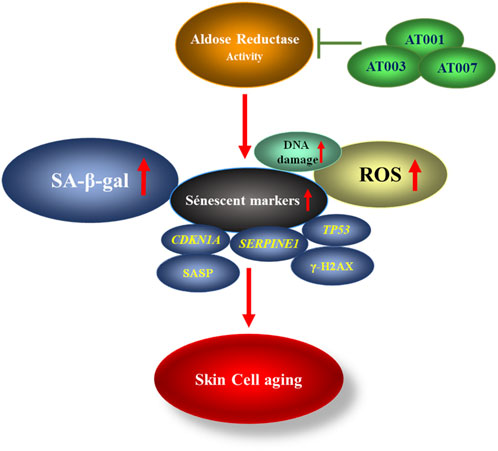
Scheme 1. Schematic representation showing AR activity increases senescent markers thereby promoting skin cell aging which can be inhibited by treatment with ARIs.
Data availability statement
The original contributions presented in the study are included in the article/Supplementary Material, further inquiries can be directed to the corresponding author.
Author contributions
GY: Conceptualization, Data curation, Formal Analysis, Methodology, Writing–original draft, Writing–review and editing. KK: Writing–review and editing, Formal Analysis. RP: Writing–review and editing, Conceptualization. SS: Writing–review and editing. AW: Writing–review and editing. RR: Conceptualization, Methodology, Supervision, Writing–original draft, Writing–review and editing.
Funding
The author(s) declare financial support was received for the research, authorship, and/or publication of this article. This work was supported by unrestricted research grant from Applied Therapeutics Inc, New York, NY, United States.
Acknowledgments
We thank Ms. Latoya Woods for the assistance with the manuscript preparation.
Conflict of interest
Authors RP, SS, and AW were employed by Applied Therapeutics Inc.
The remaining authors declare that the research was conducted in the absence of any commercial or financial relationships that could be construed as a potential conflict of interest.
The authors declare that this study received funding from Applied Therapeutics Inc. The funder had the following involvement in the study: editing the manuscript.
Publisher’s note
All claims expressed in this article are solely those of the authors and do not necessarily represent those of their affiliated organizations, or those of the publisher, the editors and the reviewers. Any product that may be evaluated in this article, or claim that may be made by its manufacturer, is not guaranteed or endorsed by the publisher.
References
Ananthakrishnan, R., Kaneko, M., Hwang, Y. C., Quadri, N., Gomez, T., Li, Q., et al. (2009). Aldose reductase mediates myocardial ischemia-reperfusion injury in part by opening mitochondrial permeability transition pore. Am. J. Physiol. Heart Circ. Physiol. 296 (2), H333–H341. doi:10.1152/ajpheart.01012.2008
Argyropoulos, A. J., Robichaud, P., Balimunkwe, R. M., Fisher, G. J., Hammerberg, C., Yan, Y., et al. (2016). Alterations of dermal connective tissue collagen in diabetes: molecular basis of aged-appearing skin. PLoS One 11 (4), e0153806. doi:10.1371/journal.pone.0153806
Chen, C., Zhou, M., Ge, Y., and Wang, X. (2020). SIRT1 and aging related signaling pathways. Mech. Ageing Dev. 187, 111215. doi:10.1016/j.mad.2020.111215
Cosgrove, M. C., Franco, O. H., Granger, S. P., Murray, P. G., and Mayes, A. E. (2007). Dietary nutrient intakes and skin-aging appearance among middle-aged American women. Am. J. Clin. Nutr. 86 (4), 1225–1231. doi:10.1093/ajcn/86.4.1225
Danby, F. W. (2010). Nutrition and aging skin: sugar and glycation. Clin. Dermatol. 28 (4), 409–411. doi:10.1016/j.clindermatol.2010.03.018
Farage, M. A., Miller, K. W., Elsner, P., and Maibach, H. I. (2008). Intrinsic and extrinsic factors in skin ageing: a review. Int. J. Cosmet. Sci. 30 (2), 87–95. doi:10.1111/j.1468-2494.2007.00415.x
Farage, M. A., Miller, K. W., and Maibach, H. I. (2017). “Determinants in the rate of skin aging: ethnicity, gender, and lifestyle influences,” in Textbook of aging skin. Editors M. A. Farage, K. W. Miller, and H. I. Maibach (Berlin, Heidelberg: Springer Berlin Heidelberg), 1709–1727.
Franco, A. C., Aveleira, C., and Cavadas, C. (2022). Skin senescence: mechanisms and impact on whole-body aging. Trends Mol. Med. 28 (2), 97–109. doi:10.1016/j.molmed.2021.12.003
Green, A. C., Hughes, M. C., McBride, P., and Fourtanier, A. (2011). Factors associated with premature skin aging (photoaging) before the age of 55: a population-based study. Dermatology 222 (1), 74–80. doi:10.1159/000322623
Gunn, D. A. (2014). “The genetics of skin aging,” in Textbook of aging skin. Editors M. A. Farage KWM,, and H. I. Maibach (Berlin, Heidelberg: Springer Berlin Heidelberg), 1–14.
Hovest, M. G., Bruggenolte, N., Hosseini, K. S., Krieg, T., and Herrmann, G. (2006). Senescence of human fibroblasts after psoralen photoactivation is mediated by ATR kinase and persistent DNA damage foci at telomeres. Mol. Biol. Cell 17 (4), 1758–1767. doi:10.1091/mbc.e05-08-0701
Hwang, J. W., Yao, H., Caito, S., Sundar, I. K., and Rahman, I. (2013). Redox regulation of SIRT1 in inflammation and cellular senescence. Free Radic. Biol. Med. 61, 95–110. doi:10.1016/j.freeradbiomed.2013.03.015
Jannapureddy, S., Sharma, M., Yepuri, G., Schmidt, A. M., and Ramasamy, R. (2021). Aldose reductase: an emerging target for development of interventions for diabetic cardiovascular complications. Front. Endocrinol. (Lausanne) 12, 636267. doi:10.3389/fendo.2021.636267
Kang, E. S., Iwata, K., Ikami, K., Ham, S. A., Kim, H. J., Chang, K. C., et al. (2011). Aldose reductase in keratinocytes attenuates cellular apoptosis and senescence induced by UV radiation. Free Radic. Biol. Med. 50 (6), 680–688. doi:10.1016/j.freeradbiomed.2010.12.021
Kaul, Z., Cesare, A. J., Huschtscha, L. I., Neumann, A. A., and Reddel, R. R. (2011). Five dysfunctional telomeres predict onset of senescence in human cells. EMBO Rep. 13 (1), 52–59. doi:10.1038/embor.2011.227
Lakdawala, N., Babalola, O., Fedeles, F., McCusker, M., Ricketts, J., Whitaker-Worth, D., et al. (2013). The role of nutrition in dermatologic diseases: facts and controversies. Clin. Dermatol 31 (6), 677–700. doi:10.1016/j.clindermatol.2013.05.004
Lee, A. Y., and Chung, S. S. (1999). Contributions of polyol pathway to oxidative stress in diabetic cataract. Faseb J. 13 (1), 23–30. doi:10.1096/fasebj.13.1.23
Lee, S. H., Lee, J. H., Lee, H. Y., and Min, K. J. (2019). Sirtuin signaling in cellular senescence and aging. BMB Rep. 52 (1), 24–34. doi:10.5483/BMBRep.2019.52.1.290
Makrantonaki, E., Bekou, V., and Zouboulis, C. C. (2012). Genetics and skin aging. Dermatoendocrinol 4 (3), 280–284. doi:10.4161/derm.22372
McDaniel, D., Farris, P., and Valacchi, G. (2018). Atmospheric skin aging-Contributors and inhibitors. J. Cosmet. Dermatol 17 (2), 124–137. doi:10.1111/jocd.12518
Mylari, B. (2014a). InventorWater soluble salts of aldose reductase inhibitors for treatment of diabetic complications. U.S. Pat. Appl. patent US20140228319, A120140814.
Mylari, B. (2014b). InventorWater soluble salts of aldose reductase inhibitors for treatment of diabetic complications. PCT Int. Appl patent WO2014126885, A120140821.
Ness, A. (2004). Diet, nutrition and the prevention of chronic diseases. WHO technical report series 916. Report of a joint WHO/FSA expert consultation. Int. J. Epidemiol. 33 (4), 914–915. doi:10.1093/ije/dyh209
Panich, U., Sittithumcharee, G., Rathviboon, N., and Jirawatnotai, S. (2016). Ultraviolet radiation-induced skin aging: the role of DNA damage and oxidative stress in epidermal stem cell damage mediated skin aging. Stem Cells Int. 2016, 7370642. doi:10.1155/2016/7370642
Ramasamy, R., and Goldberg, I. J. (2010). Aldose reductase and cardiovascular diseases, creating human-like diabetic complications in an experimental model. Circ. Res. 106 (9), 1449–1458. doi:10.1161/CIRCRESAHA.109.213447
Ramis, I., Roselló-Catafau, J., Bulbena, O., Picado, C., and Gelpi, E. (1989). 15-Hydroxyeicosatetraenoic acid as a major eicosanoid in nasal secretions: assay by high-performance liquid chromatographic-radioimmunoassay and gas chromatographic-mass spectrometric procedures. J. Chromatogr. 496 (2), 416–422. doi:10.1016/s0378-4347(00)82589-x
Ruiz, F. X., Gallego, O., Ardèvol, A., Moro, A., Domínguez, M., Alvarez, S., et al. (2009). Aldo-keto reductases from the AKR1B subfamily: retinoid specificity and control of cellular retinoic acid levels. Chem. Biol. Interact. 178 (1-3), 171–177. doi:10.1016/j.cbi.2008.10.027
Ruiz, F. X., Moro, A., Gallego, O., Ardèvol, A., Rovira, C., Petrash, J. M., et al. (2011). Human and rodent aldo-keto reductases from the AKR1B subfamily and their specificity with retinaldehyde. Chem. Biol. Interact. 191 (1-3), 199–205. doi:10.1016/j.cbi.2011.02.007
Sedelnikova, O. A., Horikawa, I., Zimonjic, D. B., Popescu, N. C., Bonner, W. M., and Barrett, J. C. (2004). Senescing human cells and ageing mice accumulate DNA lesions with unrepairable double-strand breaks. Nat. Cell Biol. 6 (2), 168–170. doi:10.1038/ncb1095
Shendelman, S. (2018). InventorAldose reductase inhibitors such as boronic acid and boronate ester compounds and uses thereof. PCT Int. Appl patent WO2018200258, A120181101.
Sibbitt, W. L., Mills, R. G., Bigler, C. F., Eaton, R. P., Griffey, R. H., and Vander Jagt, D. L. (1989). Glucose inhibition of human fibroblast proliferation and response to growth factors is prevented by inhibitors of aldose reductase. Mech. Ageing Dev. 47 (3), 265–279. doi:10.1016/0047-6374(89)90038-9
Srivastava, S. K., Yadav, U. C., Reddy, A. B., Saxena, A., Tammali, R., Shoeb, M., et al. (2011). Aldose reductase inhibition suppresses oxidative stress-induced inflammatory disorders. Chem. Biol. Interact. 191 (1-3), 330–338. doi:10.1016/j.cbi.2011.02.023
Stefanadi, E. C., Dimitrakakis, G., Antoniou, C. K., Challoumas, D., Punjabi, N., Dimitrakaki, I. A., et al. (2018). Metabolic syndrome and the skin: a more than superficial association. Reviewing the association between skin diseases and metabolic syndrome and a clinical decision algorithm for high risk patients. Diabetol. Metab. Syndr. 10, 9. doi:10.1186/s13098-018-0311-z
Tang, W. H., Stitham, J., Jin, Y., Liu, R., Lee, S. H., Du, J., et al. (2014). Aldose reductase-mediated phosphorylation of p53 leads to mitochondrial dysfunction and damage in diabetic platelets. Circulation 129 (15), 1598–1609. doi:10.1161/CIRCULATIONAHA.113.005224
Tang, W. H., Wu, S., Wong, T. M., Chung, S. K., and Chung, S. S. (2008). Polyol pathway mediates iron-induced oxidative injury in ischemic-reperfused rat heart. Free Radic. Biol. Med. 45 (5), 602–610. doi:10.1016/j.freeradbiomed.2008.05.003
Thiagarajan, D., Quadri, N., Jawahar, S., Zirpoli, H., Del Pozo, C. H., Lopez-Diez, R., et al. (2022). Aldose reductase promotes diet-induced obesity via induction of senescence in subcutaneous adipose tissue. Obes. (Silver Spring) 30 (8), 1647–1658. doi:10.1002/oby.23496
Tobin, D. J. (2017). Introduction to skin aging. J. Tissue Viability 26 (1), 37–46. doi:10.1016/j.jtv.2016.03.002
Vaughan, D. E., Rai, R., Khan, S. S., Eren, M., and Ghosh, A. K. (2017). Plasminogen activator inhibitor-1 is a marker and a mediator of senescence. Arterioscler. Thromb. Vasc. Biol. 37 (8), 1446–1452. doi:10.1161/ATVBAHA.117.309451
Vedantham, S., Ananthakrishnan, R., Schmidt, A. M., and Ramasamy, R. (2012). Aldose reductase, oxidative stress and diabetic cardiovascular complications. Cardiovasc Hematol. Agents Med. Chem. 10 (3), 234–240. doi:10.2174/187152512802651097
Vedantham, S., Thiagarajan, D., Ananthakrishnan, R., Wang, L., Rosario, R., Zou, Y. S., et al. (2014). Aldose reductase drives hyperacetylation of Egr-1 in hyperglycemia and consequent upregulation of proinflammatory and prothrombotic signals. Diabetes 63 (2), 761–774. doi:10.2337/db13-0032
Wasmuth, A., Landry, D., Deng, S., Ramasamy, R., Schmidt, A., and Mylari, B. (2013). InventorsPreparation of oxodihydropyrazinopyridazine derivatives for use as aldose reductase inhibitors. USA: PCT Int. Appl patent US20130225592, A120130829 patent U.S. Pat. Appl. Publ. US20130225592, A120130829.
Wasmuth, A., Landry, D., Deng, S., Ramasamy, R., Schmidt, A., and Mylari, B. (2014). InventorsPreparation of oxodihydropyrazinopyridazine derivatives for use as aldose reductase inhibitors. USA: PCT Int. Appl patent WO2014113380, A120140724.
Williamson, J. R., Chang, K., Frangos, M., Hasan, K. S., Ido, Y., Kawamura, T., et al. (1993). Hyperglycemic pseudohypoxia and diabetic complications. Diabetes 42 (6), 801–813. doi:10.2337/diab.42.6.801
Yepuri, G., Sukhovershin, R., Nazari-Shafti, T. Z., Petrascheck, M., Ghebre, Y. T., and Cooke, J. P. (2016). Proton pump inhibitors accelerate endothelial senescence. Circ. Res. 118 (12), e36–e42. doi:10.1161/CIRCRESAHA.116.308807
Keywords: aldose reductase, aldose reductase inhibitors, skin cell aging, senescence, oxidative stress
Citation: Yepuri G, Kancharla K, Perfetti R, Shendelman S, Wasmuth A and Ramasamy R (2024) The aldose reductase inhibitors AT-001, AT-003 and AT-007 attenuate human keratinocyte senescence. Front. Aging 5:1466281. doi: 10.3389/fragi.2024.1466281
Received: 17 July 2024; Accepted: 28 November 2024;
Published: 17 December 2024.
Edited by:
Rajarajan Amirthalingam Thandavarayan, Houston Methodist Hospital, United StatesReviewed by:
Melissa L. Harris, University of Alabama at Birmingham, United StatesMin Young Park, New York University, United States
Copyright © 2024 Yepuri, Kancharla, Perfetti, Shendelman, Wasmuth and Ramasamy. This is an open-access article distributed under the terms of the Creative Commons Attribution License (CC BY). The use, distribution or reproduction in other forums is permitted, provided the original author(s) and the copyright owner(s) are credited and that the original publication in this journal is cited, in accordance with accepted academic practice. No use, distribution or reproduction is permitted which does not comply with these terms.
*Correspondence: Ravichandran Ramasamy, cmF2aWNoYW5kcmFuLnJhbWFzYW15QG55dWxhbmdvbmUub3Jn